Preclinical studies for plant-based oral enzyme replacement therapy (Oral-ERT) in Pompe disease knockout mice with transgenic tobacco seeds expressing human GAA (tobrhGAA)
Frank Martiniuk1, Adra Mack1,3, Justin Martiniuk1,3, Gregory O. Voronin4, Shoreh Miller5, David Reimer6, Nancy Rossi7, Leslie Sheppard Bird8, Sussan Saleh8, Ruby Gupta8, Mariel Nigro8, Peter Meinke9, Benedikt Schoser9, Feng Wu10 , Angelo Kambitsis, John Arvanitopoulos1, Elena Arvanitopoulos1 and Kam-Meng Tchou-Wong11
1 JME Group, Inc, 24 Ford Lane, Roseland, NJ 07068, PsychoGenetics Center, 215 College Rd., Lab 213, Paramus, NJ 07652 JMEgroup@jmescience.com 551-999-06231, ak@jmescience.com, ya@jmescience.com, whom reprints should be directed
2 NYU School of Medicine, Department of Medicine, NY, NY 10016 frank.martiniuk@gmail.com 201-398-5085
3 shared equally in authorship and experiments, jmartin81@gmail.com, adramack@optonline.net
4 Shoreh Miller, DVM, PhD, Diplomate ACLAM Adjunct Assistant Professor, Cell Biology, Pediatric Department, RWJMS Director, In Vivo Research Services (IVRS) Rutgers University Animal Care U (RUAC) Rutgers, the State University of New Jersey Rm 017, CABM 679 Hoes Lane West Piscataway, NJ, 08854-8021 Office: 848-445-7333, Cell: 609-235-6074, shorehmiller@rutgers.edu
5 Gregory Voronin, DVM, gregoryvoronindvm@gmail.com, 908-524-4792 Associate Director, In Vivo Research Services Rutgers, The State University of New Jersey
6 David Reimer, DVM, MBA Associate Director, Veterinary Services Comparative Medicine Resources Office, 848-445-7394 reimerd@ored.rutgers.edu
7 Nancy Rossi, B.S. RLATG Animal Research Facility Supervisor Comparative Medicine Resources, Nelson Biological Laboratories Rutgers, The State University of New Jersey, 604 Allison Road, Room D-105
Piscataway, NJ 08854, (848) 445-8412-office (732) 233-2023-cell, nbrossi@ored.rutgers.edu
8 Leslie Sheppard Bird, CVT, RLATg Veterinary Technician Supervisor Comparative Medicine Resources Rutgers, The State University of NJ, D108 Nelson Biological Laboratories, 604 Allison Road Piscataway, NJ 08854-8082, Main Line: 848-445-4168 Direct Line: 848-445-7365. sheppale@ored.rutgers.edu, Ruby Gupta rg529@ca.rutgers.edu, Mariel Nigro, mn496@ored.rutgers.edu, Sussan Saleh, ss868@ored.rutgers.edu
9 Friedrich-Baur-Institute, Department of Neurology, LMU Klinikum,, Ludwig-Maximilians-University, Munich, 1, 80336 München, Germany +49 89 4400 57400 Peter.Meinke@med.uni-muenchen.de, Benedikt.Schoser@med.uni-muenchen.de
10 NYU Langone Medical Center, Department of Population Health, Smilow Research Center 1206, 522 First Avenue, NY 10016, 212-263-9226, feng.wu@nyulangone.org
11 Icahn School of Medicine at Mount Sinai, Ronald M. Loeb Center for Alzheimer's Disease, 1425 Madison Avenue, New York, NY 10029, 212-659-5671, kammengtchouwong@gmail.com
Key words Recombinant human acid maltase, Transgenic tobacco plants, Pompe disease, Oral enzyme replacement
Abbreviations AMD = acid maltase deficiency; rhGAA = recombinant GAA: tobrhGAA = recombinant GAA produced in tobacco seeds; GAA = acid maltase; ERT = enzyme replacement therapy; Oral-ERT = oral enzyme replacement therapy;
ABSTRACT
Genetic deficiency of acid a-glucosidase (GAA) results in glycogen storage disease type II (GSDII) or Pompe disease encompassing at least four clinical subtypes of varying severity (infantile; childhood, juvenile and late onset). Our objective is to develop an innovative and affordable approach for enzyme replacement therapy (ERT) via oral administration (Oral-ERT) to maintain a sustained, therapeutic level of enzyme on a daily basis to improve efficacy of treatment and quality of life for people living with Pompe disease. A consensus at a 2019 US Acid Maltase Deficiency (AMDA) conference suggested that a multi-pronged approach including gene therapy, diet, exercise, etc. must be evaluated for a successful treatment of Pompe disease. Tobacco seeds contain the metabolic machinery that is more compatible with mammalian glycosylation-phosphorylation and processing. Previously, we have shown that a lysate from transgenic tobacco seeds expressing human GAA (tobrhGAA) was enzymatically active and can correct enzyme deficiency in cultured deficient-cells and in adult lymphocytes of Pompe patients and in vivo in disease-relevant tissues in GAA knockout (KO) mice when administered IP.
We have extended these pre-clinical studies in Pompe knockout (KO) mice with ground tobrhGAA seeds that supports proof-of-concept for Oral-ERT for future clinical trials. Briefly in GAA KO mice, Oral-ERT with ground tobrhGAA seeds showed significant reversal of fore-limb and hind-limb muscle weakness, increased motor coordination/balance/strength and mobility, improved spontaneous learning, increased GAA baseline activity in tissues, reduced glycogen in tissues and negible serum titers to GAA. Pharmacokinetics showed maximum serum GAA concentration (Cs) at 8-10 hr and peak urine excretion at 10-12 hr. The tobrhGAA was taken up in PD fibroblast, lymphoid and myoblast cells of Pompe patients. Enzyme kinetics compared favorably or superior to placental hGAA, plus alglucosidase alfa or other rhGAAs for Km, Vmax, pH optima, thermal heat stability and IC50 for inhibitors. The tobrhGAA in seeds was extremely stable stored for 15 years at room temperature. NGS-genome sequencing of the tobrhGAA and wild-type plants and RNA expression profiles was performed and will be posted on our website. Thus, Oral-ERT with ground tobrhGAA seeds is an innovative approach that overcomes some of the challenges of alglucosidase alfa-ERT and provides a more effective, safe and significantly less expensive treatment.
INTRODUCTION
Genetic deficiency of the lysosomal acid maltase or acid a-glucosidase (GAA) results in acid maltase deficiency (AMD) or Pompe disease encompassing at least four clinical subtypes of varying severity (infantile; childhood, juvenile and adult onset)(1). Pompe disease exhibits intracellular accumulation of glycogen in multiple tissues with skeletal muscle being the primary target, manifesting as myopathy and cardiomyopathy (2-5). The classic infantile form exhibits no enzyme activity, muscle weakness, feeding difficulties and hypertrophic cardiomyopathy, leading to death in the first year (6-8). The adult-onset form has partial enzyme deficiency, manifesting with slowly progressive muscle weakness leading to wheelchair/ventilator dependence and premature death from ventilator insufficiency (9-11). The incidence of people living with Pompe disease is estimated at 1:40,000 (12)(Orpha number-ORPHA365 at www.orpha.net). Recently, Park (13) re-calculated the carrier frequency (pCF) and predicted genetic prevalence (pGP) using general population databases based on the proportion of causative genotypes. Total pCF and pGP were 1.3% (1 in 77) and 1:23,232. The highest pGP was in the E. Asian population at 1:12,125, followed by Non-Finnish European (1:13,756), Ashkenazi Jewish (1:22,851), African/African-American (1:26,560), Latino/Admixed American (1:57,620), S. Asian (1:93,087) and Finnish (1:1,056,444). Unfortunately, the number of people in each population enrolled may not reflect the real-world population.
ERT with a recombinant human GAA (rhGAA) secreted by CHO cells (alglucosidase alfa/Myozyme/Lumizyme, Genzyme/Sanofi Corp.) infused every two weeks is the first approved therapy. Although it is efficient in rescuing cardiac abnormalities and extending the life span of infants, the response in skeletal muscle is variable (marketed dose of 20 mg/kg/every 2 weeks-iv) via the cation-independent mannose 6-phosphate receptor (14-17). In infants, muscle pathology and degree of glycogen deposition correlates with the severity of symptoms and the earlier ERT is introduced, the better chance of response (14). In adult-onset patients, mild improvements in motor and respiratory functions have been achieved which is unsatisfactory in the reversal of skeletal muscle pathology (14-17). In adult-onset patients, the ability for muscle to metabolize extra-lysosomal as well as intra-lysosomal glycogen is impaired. Lysosomal glycogen accumulation leads to multiple secondary abnormalities (autophagy, lipofuscin, mitochondria, trafficking and signaling) that may be amenable by long-term therapy. ERT usually begins when the patients are symptomatic and these secondary problems are already present contributing to inefficient delivery/uptake of alglucosidase alfa to muscle (18). The outcome of infantile patients is determined by many factors, among which are age, disease severity at ERT, genotype, genotype dependent CRIM status and the height of the antibody response. High and sustained antibody titers need to be prevented to achieve a good response to ERT, however titers varied substantially between patients and does not strictly correlate with the patients’ CRIM status. Only infantile patients can be either CRIM-negative or CRIM-positive. Since all juvenile and adult patients make at least some amount of residual GAA, they are always CRIM-positive. About 40% of infantile patients are CRIM-negative while the remaining 60% are CRIM-positive. The immune response may be minimized by early start of ERT and pretreatment by an immune tolerance induction regime using e.g. a combination of rituximab, methotrexate, bortezomib and IV immunoglobulins. Up to 20% of adult patients develop high titers on ERT, 40% intermediate and 40% none or low titers (19-40). In adults antibody formation does not interfere with rhGAA efficacy in the majority of patients, however may be associated with infusion-associated reactions (IARs) and may be attenuated by the IVS1/delex18 (c.2481+102_2646+31del, expression of a truncated protein) GAA genotype (41). Some patients experienced IARs due to bronchial spasm with flushing and pulmonary artery blockage during infusion. Several studies suggest that unchanged endogenous GAA protein expression lowers the chance of forming antibodies to recombinant proteins. Gutschmidt et al. (42) found that ERT in late onset patients’ clinical outcomes, particularly lung function, muscle strength and walking capability tend to deteriorate over time, indicating that additional efforts must be made to improve ERT effectiveness and supplement therapies developed. Although alglucosidase alfa has been a wonderful first step in treating people with Pompe disease, it has revealed subtle aspects that must be dealt with for successful treatment (18,42-48). ERT has unmasked previously unrecognized clinical manifestations include tracheo-bronchomalacia, vascular aneurysms and GI discomfort that impacts smooth muscle. Persistent smooth muscle pathology has a substantial impact on quality of life and leads to life threatening complications. In addition to airway smooth muscle weakness, vascular deterioration, GI discomfort and loss of genitourinary control have been observed. Cerebral and aortic aneurysms have caused microhemorrhages leading to symptoms ranging from headaches and numbness to coma and death (49-55). Pompe disease results in subsequent pathology in smooth muscle cells may lead to life-threatening complications if not properly treated (56). In GAA KO mice, there is increased glycogen in smooth muscle cells of the aorta, trachea, esophagus, stomach and bladder plus increases in lysosome membrane protein (LAMP1) and autophagosome membrane protein (LC3). More importantly, lifelong treatment ($250-500k/yr/adult) can be prohibitively expensive resulting in the reluctance of insurance companies to reimburse costs for adult patients (57) underlining the demand for more economical production and novel delivery strategies for treatment. A consensus at a 2019 US Acid Maltase Deficiency Association conference in San Antonio, TX, suggested that a multi-pronged approach including gene therapy, diet, exercise, etc. must be evaluated for a successful treatment of Pompe disease.
The technological platform involving the accumulation of recombinant proteins in seeds warrants better availability of the products and allows long-term storage of the biomass for processing (58-60). Transgenic plants, seeds and cultured plant cells are potentially one of the most economical systems for large-scale production of recombinant enzymes for pharmaceutical uses (60-68). Seeds are particularly attractive due to their high rates of protein synthesis and their ability to remain viable in the mature-dry state as a stable repository (67-80). Over one-third of approved pharmaceutical proteins are glycoproteins (72-82) and even minor differences in N-glycan structures can change the distribution, activity or longevity of recombinant proteins when compared with their native counterparts, altering their efficacy as therapeutics (59,60,83-86). Protalix Biotherapeutics and Shaaltiel et al. (86,87) produced a glucocerebrosidase for ERT of Gaucher disease using a plant cell system and an Exploratory, Open-label Study to Evaluate the Safety of PRX-112 and PK of oral prGCD in patients receiving 250 ml of a re-suspended carrot cells. Administration of PRX-112 using carrot cells as carrier vehicle, may overcome absorption, degradation and uptake in the small intestine and can be found in the blood stream in an active form (88-90).
Previously, we expressed the human GAA cDNA in chloroplasts, callus and leaves of transgenic tomato and tobacco plants but was not enzymatically active. The hGAA was expressed in tobacco seeds (tobrhGAA) by targeting to the ER using the signal peptide sequence/promoter of soybean β-conglycin (91). The tobrhGAA from transgenic plant seeds was enzymatically active, taken up by Pompe disease fibroblasts and WBCs to reverse the enzyme defect and binds to the affinity matrix Sephadex G100 and eluted by maltose. A crude lysate of transgenic seeds administered intraperitoneally in GAA KO mice increased GAA activity to 10-20% of normal in tissues, most notably heart, skeletal muscle and diaphragm (91-96).
In this report, we have extended these pre-clinical studies in Pompe disease KO mice with ground tobrhGAA seeds that supports proof-of-concept for Oral-ERT for people living with Pompe disease for future clinical trials. Briefly in GAA KO mice, Oral-ERT with various preparations of tobrhGAA showed significant reversal of fore-limb and hind-limb muscle weakness, increased motor coordination/balance/strength and mobility, improved spontaneous learning, increased GAA baseline activity in tissues, reduced glycogen in tissues and negligible serum titers to GAA. Pharmacokinetics showed maximum serum GAA concentration (Cs) at 8-10 hr and peak urine excretion at 10-12 hr. The tobrhGAA was taken up in fibroblast and lymphoid cells from infantile, juvenile and adult onset patients comparable to human placenta GAA and an rhGAA. Exposure of tobrhGAA to a human Pompe myoblast cell line increased GAA to 24-35% of normal. Enzyme kinetics for tobrhGAA vs placental hGAA, plus alglucosidase alfa or other rhGAAs for Km, Vmax, pH optima, thermal heat stability and IC50 for inhibitors and our tobrhGAA is comparable to placental GAA and superior to alglucosidase alfa and other rhGAAs. The tobrhGAA in seeds stored for 15 years at room temperature showed less than 15% loss in GAA activity indicating extreme stability. NGS-genome sequencing of the tobrhGAA and wild-type plants and RNA/proteome expression profiles will be posted on our website (under construction). Oral-ERT with tobrhGAA is an innovative approach that overcomes some of the challenges of alglucosidase alfa-ERT and provides a more effective, safe and less expensive treatment. This protocol will be the second oral ERT plant-made pharmaceutical (PMPs)(83,88-90,95). MATERIAL and METHODS
Hydroponic Growth of Tobacco Plants Transgenic tobacco plant#3 expressing human GAA in seeds are grown indoors using a hydroponic system (Active Aqua Grow Flow Kit, Hydrobuilder, Inc.) with deionized water (eliminating water/soil contaminants). Seed-pods are harvested and dried in freeze-dryer followed by separation from the husk by passage through a standard food mesh strainer. To eliminate environmental contamination, seeds were ground for 10 minutes in a porcelain pestle-mortar until a fine powder and placed in a UV germicidal incubator for 30 minutes to sterilize. Viability of 0.1 grams (~1,000 whole seeds) are grown in a 150 mm petri dish on paper towels saturated with water for 7-8 days at room temperature.
Simulated Stomach and Small Intestinal Environments To mimic the stomach and small intestine (SI) environment, we exposed the 100 mg of whole or milled tobrhGAA seeds to physiologic conditions/times to pepsin (stomach) followed by trypsin/chymotrypsin (small intestine)(87,96). Samples were added to 300 mg/ml pepsin at pH 4.0 for 60 min., adjusted pH to 6.5 and then trypsin at 800 mg/ml and chymotrypsin at 700 mg/ml for 60 min at 37oC followed by GAA assay.
Enzyme Assay Ground seeds or tissues were resuspended in 10 mM sodium phosphate, pH 7.5, frozen and thawed 3x and centrifuged at 13,000g for 10 min. The supernatants were assayed for GAA using the artificial substrate 4-methylumbelliferyl-a-D-glucoside (4-MU-Glc, 1 mg/ml) at pH 4.0 (0.5 M sodium acetate) and as an internal control, neutral alpha glucosidase (NAG) at pH 7.5 (0.5 M sodium phosphate) for 18 hours. Fluorescence was determined in a fluorometer (excitation-360nm and emission-460nm) (Sequoia-Turner) as previously described (97).
Biochemical/Enzyme Kinetic Analyses We compared a lysate of tobrhGAA seeds to a rhGAA (R&D Systems) and mature placental human GAA for specific activity using 4-MU-Glc at pH 4.0, maltose and glycogen, pH optima, inhibitors (acarbose, castanospermine, deoxynojirimycin, miglitol, voglibose) and heat stability (94,98-108) using standard enzyme kinetic methods.
Uptake of tobrhGAA by Pompe Disease Human Myoblast, Fibroblast and Lymphoid Cell Lines Human lymphoid (GM6314, GM13793, GM14450) or fibroblast (GM4912, GM1935, GM3329) cell lines from infantile or adult Pompe disease were maintained in 15% fetal bovine serum, RPMI 1640 or DMEM supplemented with glutamine, penicillin and streptomycin at 37oC-5% CO2. Cells were plated at 0.3-0.4 x 106/6-well in 1.5 ml media-24 hr before addition of varying amounts of tobrhGAA or others GAA formulations (92). Cells were harvested after various hours of exposure, washed with PBS, lysed by addition of 0.5 ml of 0.01 M sodium phosphate pH 7.5, frozen and thawed 3x, spun for 5 minutes to clarify and assayed for human GAA and NAG as described above. A human PD myoblast cell line (homozygous for the IVS1 c.-32–13t>g)(109) and normal skeletal muscle cells were plated at 0.3 to 0.4 x 106 in a 6-well plate with PromoCell skeletal muscle growth media. Cells were exposed a lysate of tobrhGAA for 48 hr and assayed. Mock treated GAA and normal myoblast cells were controls plus cells treated with equivalent amounts of a rhGAA (R&D Systems #8329-GH-025). Simultaneously, we measured cell proliferation with the Cayman MTT cell proliferation assay (#10009365).
Short-term Studies GAA KO Mice We utilized the GAA KO mouse with the exon 6neo disruption (110), wild-type BALB/c or 129/J or GAA KO mice mock-treated with PBS. GAA KO mice (~4-6 months old) were given oral administrations every other day to day 7 a lysate from 300 mg (~75 mg tobrhGAA) of transgenic seeds mixed 3:1 with apple juice as a safe, non-invasive oral administration technique (111) and grip-strength measured by a grip-strength meter (GSM)(Columbus Inst. OH). At day 7, mice were sacrificed and tissues assayed for GAA and NAG and compared to wild-type mice and mock (PBS) treated GAA KO mice.
Long-term Treatment by Oral Gavage in GAA KO Mice We treated two groups (age matched) of GAA KO mice (exon 6neo)(n=3) three times weekly with either a 1x or 3x dose (containing either 25 or 75 mg tobrhGAA per dose) mixed 3:1 with apple juice as a safe, non-invasive oral administration technique (100) and measured vertical hang-time as performed by Raben et al. (110).
Oral-ERT in GAA KO Mice GAA KO mice were fed either 50 mg or 150 mg tobrhGAA ground seeds-daily mixed with peanut butter in petri dishes (12:12 L/D photoperiod)(91)(age ~5 months)(B6,129-Gaatm1Rabn/J mice, 110). Tissues, urine, blood slides, weight and serum were collected. Mice were tested every 3 weeks for: motor activity by running wheel (RW-Mini-Mitter Co., Inc., OR); fore-limb muscle strength by grip-strength meter (GSM)(Columbus Inst. OH), motor coordination/balance with a Rotarod (AJATPA Expert Industries, India), open-field mobility by a 5 minute video for distance traveled and spontaneous alternative learning with a T-maze (Stoelting, IL).
ELISA for Antibodies to tobrhGAA In Falcon plates #3912, add Sephadex G100 purified human placental GAA (112) at 1 mg/well in 100 ml PBS at 4oC overnight, wash 3x with PBS, block with 200 ml PBS-1g% BSA-0.05% Tween-20 for 60 min. or longer at RT. Add 1 ml serum in 100 ul PBS-overnight at 4oC. Wash 3x PBS, 1x PBS-0.05% Tween-20, 2X PBS. Add 100 ml goat anti-mouse IgG-HRP (BioRad #172-1101) at 1:500 in PBS/BSA/Tween-20 at 4oC overnight. Wash 3x PBS, 1x PBS-0.05% Tween-20, 2x PBS. Stain with 100 ml of 3,3’,5,5’–tetramethylbenzidine (Sigma #T5525) in 9 ml of 0.05 M phosphate-citrate buffer, pH 5.0 (Sigma #P4922) with 20 ml of 30% H2O2 for 30-60 minutes at room temperature. Reactions are stopped with 50 ml 1 N HCl and read at A450nm.
Endotoxin Determination Endotoxin in various amounts of a lysate of tobrhGAA seeds was determined using the Pierce LAL Chromogenic Endotoxin Quantitation Kit (#88282) according to manufacturers instructions (113).
Bradford protein assay 150 ul reagent (BioRad #500-0006) diluted 1->5 with water in a 96 well microtiter plate. Standards were serial dilutions of BSA from 1,000 mg/ml to 2 mg/ml and 1 to 25 ml samples. Samples were read at A595nm before 60 min.
Assay for Glycogen Content In a 96-well microtiter plate, add 25 ml sample, 25 ml 0.05 M sodium phosphate pH 6.5, +0.5 ml amyloglucosidase (Sigma #A-1602), placed overnight at 37oC (114). Glycogen standards (rabbit liver, 2 mg/ml) and D-glucose standards were at 400 mM, 200 mM and 100 mM. Add 20 ml of Eton Bioscience glucose assay solution (#1200031002) and incubate for 30 minutes at 37°C. The reaction was stopped with 25 ml of 0.5 M acetic acid and read at A490nm.
Ames Mutagenicity Test on E.coli DH5aand DH5apUC19 plated on NZ media with ampicillin and various amounts of tobrhGAA lysate and colony forming units counted (113,115-118).
CBC differentials CBC differentials were perform on blood smears on slides with Giemsa-Wright staining.
Extracton of DNA and RNA from Seeds for NGS and RNA Transcriptome profile DNA and total RNA was extracted from wild-type (Nicotiana tobacum L. cv. xanthi) and tobrhGAA seeds by kits (Thermo Scientific GeneJET Plant RNA purification kit-K0801; GeneJET Plant DNA purification kit-K0791). The NGS-genome sequence and RNA expression profiles was performed at BGI.com and global proteomic profiling by UPLC-MS/MS at bioproximity.com.
Nicotine Levels in Leaves and Seeds The levels of nicotine in tobrhGAA#3 seeds and leaves was measured by gas chromatography-mass spectroscopy (GC/MS) at Avogado nAnalytical, LLC, Salem, NH.
Statistical Analysis Student t-test for probability associated with a population and standard deviation based upon the population were determined using Microsoft Excel software and considered significant at p = ≤ 0.05.
RESULTS
We have extended our original studies in the production of a functional human acid maltase in tobacco seeds: biochemical analysis, uptake by human Pompe disease cells, and in vivo studies in GAA knockout mice (91) that is organized into three categories-A-Miscellaneous studies, B-in vitro studies and C-in vivo studies which evolved from the use of whole seeds or seed lysates to the final ground seeds.
A-Miscellaneous Studies
A.1 Hydroponic growth of tobacco plants and processing of seeds Transgenic tobacco plant #3 expressing human GAA in seeds are grown indoors using a hydroponic system with deionized water (eliminating water/soil contaminants). Seed-pods are harvested and dried in freeze-dryer followed by separation from husk through a standard food mesh strainer. To eliminate environmental contamination, ground seeds were tested for lack of viability and germination. Seeds are grounded for 10 minutes in a porcelain pestle-mortar until a fine powder followed by UV sterilization. Approximately, 0.1 gram of ground/sterilized seeds (~1,000 whole seeds) and untreated transgenic seeds are germinated (positive control) by evenly distributed in a petri dish on paper towels saturated with water and observed for 7-8 days at room temperature. Viable seeds will germinate in a week (Fig. 1). No germination was observed in processed seeds.
A.2 Nicotine levels in leaves and seeds was measured by GC/MS (Avogado nAnalytical, LLC, Salem, NH) and contained <5ng/dry gram of tobrhGAA seeds or leaves and generated a GC/MS spectral profile for future comparisons.
A.3 Long-term stability We compared tobrhGAA in seeds stored for 9 and 15 years at room temperature vs. freshly harvested seeds. We found that there was less than 15% loss in GAA activity between the seeds indicating extreme stability (old = 0.20 mg tobrhGAA/g seeds vs. fresh-0.25 mg tobrhGAA/g seeds) for both age groups.
A.4 WGS, RNA and proteomic profiles The WGS genome sequence and RNA expression profiles of the tobrhGAA#3 and wild-type plants were performed at BGI.com and global proteomic profiling by UPLC-MS/MS at bioproximity.com. Proteomic profile showed 285 proteins expressed in wild-type and tobrhGAA#3 seeds. Besides the hGAA, ~20 proteins were found differentially expressed in tobrhGAA#3 seeds. Once the analyses and comparisons are completed, the data will be shared and posted on our website (under construction).
A.5 Endotoxin levels in an extract of tobrhGAA#3 seeds with an LAL endotoxin kit was less than 0.25 EU/ml endotoxin or ~25 pg/ml, values much lower than the estimate of 300 mg/kg for oral endotoxin toxicity in mammals and humans (119-121). We also found no viable anerobic or aerobic bacteria.
A.6 Affect of stomach and small intestinal environments To mimic the stomach and small intestine (SI) environments, we exposed the tobrhGAA to physiologic conditions and times to pepsin (stomach) and trypsin/chymotrypsin (small intestine)(87,96). Lysosomal GAA is stable at low pH. We exposed a lysate from tobrhGAA#3 seeds to 300 mg/ml pepsin at pH 4.0 followed by trypsin at 800 mg/ml-pH 6.5/chymotrypsin at 700 mg/ml-pH 6.5 for 60 min at 37oC. None of the enzymes had any affect on tobrhGAA activity, thus demonstrating that the conditions in the digestive tract probably does not affect tobrhGAA. We repeated conditions with 100 mg of whole or milled seeds. Interestingly, the amount of tobrhGAA in whole and milled seeds (600 mg/g seeds) was more than double physical lysing suggesting that the acid environment in the stomach more thoroughly disrupts and releases tobrhGAA from the whole or milled seed. These data are extremely important for future production, manufacturing, costs and availability via a pill containing whole or milled seeds.
A.7 Ames mutagenicity test was performed on E. coli DH5aand DH5apUC19 with various amounts of tobrhGAA seed lysate. We found no additional bacterial or plasmid revertant CFUs generated with a tobrhGAA lysate by exposure to >104 bacteria (113,117,118,123,124).
B-in vitro Studies
B.1 in vitro studies in Pompe disease fibroblast and lymphoid cell lines We evaluated tobrhGAA uptake in fibroblast and lymphoid cells from infantile, juvenile and adult onset patients and compared to human placenta GAA and an rhGAA (R&D Systems). Various equivalent concentrations and time-points (2, 24 and 48 hr) showed similar uptake and increases in GAA (mean+SEM)(Graphs 1 and 2).
B.2 Uptake of tobrhGAA in a human Pompe disease myoblast cell line A human Pompe disease myoblast cell line (homozygous for the IVS1 c.-32–13t>g)(109) was exposed to equivalent amounts of a lysate of tobrhGAA seeds for 48 hr and assayed. Mock treated GAA and normal skeletal muscle cells were controls plus cells treated with rhGAA. We found that tobrhGAA increased GAA to 24-35% of normal (mean+SD)(Graph 3). Simultaneously, we measured cell proliferation with the Cayman MTT assay and found that both tobrhGAA doses slightly reduced cell growth by <10%.
B.3 Biochemical/enzyme kinetic analyses We compared the enzyme kinetics fora lysate of tobrhGAA vs placental human placental GAA and an rhGAA (R&D Systems), plus limited published data for alglucosidase alfa or other rhGAAs (100-108) for Km, Vmax, pH optima, thermal heat stability and IC50 for inhibitors (acarbose, castanospermine, deoxynojirimycin, miglitol, voglibose). Data showed that showed our tobrhGAA is comparable to placental GAA and superior to alglucosidase alfa and other rhGAAs (Table 1)(94,98-108,112,124,125).
C- in vivo Studies
C.1 Short-term treatment Fore-limb grip strength was measured by a grip strength meter in GAA KO mice (exon 6neo)(110) after three oral administrations every other day to day 7 with a lysate (1x dose containing 75 mg tobrhGAA) of tobrhGAA seeds. Wild-type mice (n=3) averaged 245+21 lbs (SEM) grip at release. Mock treated GAA KOmice (n=3) averaged 92+3 lbs grip at release (3-5 attempts/mouse) and treated GAA KO mice (n=3) averaged 105+3 lbs grip at release (p = <0.024 treated vs. mock treated) with a 14% improvement in strength. At day 7, mice were sacrificed and tissues were assayed for GAA/NAG and compared to mock-treated GAA KO and wild-type mice. Importantly, we found increases in GAA activity in tissues from 8 to 23% of wild-type mice (heart, skeletal muscle, liver and diaphragm) from treated GAA KO mice (Table 2).
C.2 Long-term (203 days) treatment We treated two groups of GAA KO mice (110)(n=3)-3x weekly with either a 1x or 3x dose (25 or 75 mg tobrhGAA/dose) orally (100) and measured vertical hang-time over 203 days. The treatment was well tolerated as mice gained weight during the treatment. Both treatment groups showed a steady significant improvement in vertical hang-time vs mock treated GAA KO mice. Interestingly, mice in the 1x group gradually increased hang-time equal to the 3x dose group, suggesting that the lower dose can be effective in long-term treatment. Both treatment groups showed 25-35% vs. mock treated wild-type mice at day 82, but by day 203, both groups were almost equal (89-95%) to mock treated wild-type mice (Fig. 2).
Assay of tissues at 203 days treatment We found increases in GAA activity in tissues (heart, hind-limb skeletal muscle, kidney, liver and diaphragm) from GAA KO mice treated with tobrhGAA by oral gavage, thereby supporting Oral-ERT with tobrhGAA. The % of wild-type ranged from 13 to 38% depending upon tissue (Table 3).
Glycogen reduction in tissues We measured glycogen levels in tissues (110) after 203 days treatment and found it ranged from 18 to 48% reduction depending upon tissue (Table 4).
Immune response Serum antibody titer to oral tobrhGAA at 203 days was undetectable at 4 weeks, barely detected at a 1:10 at 8 weeks for both 1x and 3x doses and detected at 1:20, thus demonstrating low immune response (101). We simultaneously generated mouse antibody to human placental GAA by s.c. injection of 15 ug over 3 months and found the titer to be 1:1500. Thus, oral tobrhGAA of similar quantity of antigen did not generate a high serum immunoreaction.
Taken up by a distant tissue We determined if the tobrhGAA was taken up by a distant tissue-tail without sacrificing the mice and assayed for GAA at 45 and 203 days. Wild-type mice had GAA activity of 0.46+0.14, mock treated GAA KO had 0.064+0.021 and treated GAA KO had 0.10 to 0.2+0.05 for 1x or 3x dose (p=<0.006 treated vs mock treated)(Graph 4).
C.3 Treatment with whole seeds and running wheel activity GAA KO mice (3 females- ~4 months age) were fed twice daily with 50 mg whole tobrhGAA seeds and running wheel (RW) activity monitored for 21 days, followed by 50 mg-1x daily for 14 days and then 50 mg every 2 days (Fig. 3). Historical levels for wild-type and GAA KO mice and GAA KO mice feed 50 mg of wild-type tobacco seeds are indicted. GAA KO mice treated 2x daily with 50 mg tobrhGAA seeds showed increased running wheel activity to 60% of wild-type by day 24. At day 25, mice were feed 50 mg-1x daily, resulted in a reduction of RW to 40% of wild-type and at day 41, mice were feed 50 mg every other day-reduced activity to mock treated levels.
C.4 Assessment of spontaneous alternation in wild-type and GAA KO mice Spontaneous alternation is used to assess the cognitive ability of rodents to choose one of the 2 goal arms of the T-maze. The advantage of a free choice procedure is that hippocampal or lesioned animals often develop a side preference and scores below 50%. Controls generally achieve at 60-80% correct alternation. We assessed spontaneous alternative learning for cognitive ability in the T-maze in both male and female GAA KO mice and wild-type-129/C57 mice from 2-9 months of age. We found that deficiency in spontaneous learning appeared by 2-3 months in male and 3-4 months in female GAA KO mice (Graph 5). All conditions were significant (p=<0.05).
C5. Oral-ERT with tobrhGAA in GAA KO mice GAA KO mice were administered 50 mg or 150 mg tobrhGAA ground sterile seeds-daily (age 5 months)(B6,129-Gaatm1Rabn/J mice,110). Tissues, urine, blood slides, weight and serum were collected. Mice were tested every 3 weeks for: motor activity by running wheel (RW); fore-limb muscle strength by grip-strength meter (GSM), motor coordination/balance with a rotarod, open-field mobility by 5 min video/distance traveled and spontaneous learning with a T-maze. Reversal of muscle weakness showed that young mice responded faster than older mice and older mice required higher levels of tobrhGAA. Both age groups and doses showed improvement in spontaneous alternation by T-maze after 2-3 weeks (Table 5). CBC differentials of treated GAA KO animals were identical to pre-treatment GAA KO and wild-type-129/C57 mice.
C6. Steady-state GAA levels in treated tissues We measured steady-state GAA/NAG from the mice in above. Interestingly and not unexpected since the tobrhGAA is given orally, the SI sections contained the highest ratio and higher levels than wild-type-129/C57 (Table 6)(126-136).
C7. Pharmacokinetics and bio-distribution of Oral-ERT in GAA KO mice Plasma and urine clearance rate with a 3x-dose (75 mg tobrhGAA) or whole seeds in GAA KO mice at 0, 1, 2, 4, 6, 8, 10, 12, 24 and 48 hr. TobrhGAA activity showed maximum serum GAA concentration (Cs) at 8 to 10 hr and peak urine excretion at 10 to 12 hr (Fig. 4 and 5). Bio-distribution (BD) in organs (skeletal muscle, heart, diaphragm, liver, spleen, brain, lung, sectioned SI and kidney) showed different levels and times of accumulation with showed greatest levels between 12, 24 and 48 hr (not shown)(98,122,137-140).
C8. Maximum tolerated dose We determined the lethal dose in GAA KO mice (n = 4) starting at 10 g-seeds/mouse, doubling daily to 320 g. No weight loss or deaths were observed and CBCs remained unchanged.
DISCUSSION
Currently, there is no effective treatment or cure for people living with Pompe disease. Sanofi-Genzyme, Inc. using a rhGAA (alglucosidase alfa) secreted from a CHO cell line has demonstrated moderate success in patients (27), however yearly costs are very high. Although alglucosidase alfa has been a wonderful first step in treating Pompe disease it has revealed subtle aspects that must be dealt with for successful treatment (18,42-48). ERT usually begins when the patients are symptomatic, however, all the secondary problems are already present which are further compounded by issues with alglucosidase alfa uptake in muscle (Table 7)(18). Lysosomal enzymes (such as GAA) are targeted to the lysosome by a mannose-6-phosphate recognition sequence that is exposed by posttranslational modification in the Golgi that may be the mechanism that extracellular GAA can be recycled and targeted back to the lysosomes. This mechanism will potentially allow rhGAA to be delivered to the cells or tissues and directed to the lysosome. However, some GAA may be taken up or recycled by endocytosis or a mannose-6-phosphate independent mechanism (22-25). In skeletal muscle there are a low abundance of the cation-independent mannose 6-phosphate receptors (CI-MPR) compounded by low blood flow plus low affinity of the CHO-produced alglucosidase alfa for the CI-MPR. Alglucosidase alfa is delivered to the lysosome by receptor-mediated endocytosis after binding of the M6P-glycan to the CI-MPR. Alglucosidase alfa has one M6P/enzyme (141-143) and 1,000-fold lower affinity (144). With alglucosidase alfa, the high level of infused enzyme is transient, leaving the patients with no therapeutic enzyme for the remaining 13 days. Thus, oral formulations of ground tobrhGAA seeds will allow patients to ingest daily in single or multiple doses conveniently at home to sustain a therapeutic dose of enzyme activity and to improve the quality of life.
Investigators are studying the role of autophagy (18,145-147), an intracellular system for delivering portions of cytoplasm and damaged organelles to lysosomes for degradation/recycling in PD and the reduction of glycogen in skeletal muscle. A GAA and glycogen synthase 1 (GYS1) KO mouse exhibited a profound reduction of glycogen in the heart and skeletal muscles, a decrease in lysosomal autophagic build-up and correction of cardiomegaly. The abnormalities in glucose metabolism were corrected in the double-KO mice that demonstrate long-term elimination of muscle glycogen synthesis leads to a significant improvement of structural, metabolic and functional defects and a new perspective for treatment of Pompe disease (17). Lim et al. (147) reactivated mTOR in Pompe disease mice by TSC knockdown resulted in the reversal of muscle atrophy, removal of autophagic buildup plus aberrant mTOR signaling can be reversed by arginine alone (146-148). Jung et al. (149,150) produced and characterized a rhGAA in a transgenic rice cell suspension culture with high-mannose glycans and was similar to the CHO derived hGAA.
Seeds may be a better vehicle for Oral-ERT for lysosomal diseases, such as Pompe disease, compared to intravenous systemic delivery. Seeds and not other plant tissues and organelles, contain the metabolic machinery necessary for correct glycosylation, processing, phosphorylation and synthesis of complex enzymes and proteins, enzymes or proteins plus are protected or shielded from digestion in the stomach and small intestine and can be administered daily in single and multiple doses and seeds provide long-term stable storage of recombinant enzymes. A number of biotechnology companies have tried to mass produce a rhGAA from different platforms. A consensus at a 2019 US Acid Maltase Deficiency conference at San Antonio, TX, suggested that a multi-pronged approach including gene therapy, diet, exercise, etc. must be evaluated for a successful treatment of Pompe disease. Prompted by studies of oral formulations of edible tissues in broccoli sprouts, corn/maize, pea, rice, tobacco and tomato for pharmaceutical applications including vaccination to induce effective mucosal immune tolerance and immune reactions, GI, bacterial/viral infections, allergies, asthma, diabetes, endocrine-associated diseases, hypersensitivity, elevated BP, cholera, leucopenia, cancer and RA (119,151-170), we hypothesized that rhGAA from edible plant tissues offers an cost-effective, innovative and safe strategy for Oral-ERT for Pompe disease. We investigated the potential of genetically engineered “edible plant tissues” as an alternative large-scale production system that overcomes the high cost of producing rhGAA in CHO cells. Oral-ERT can be safer than infusion and be ingested in a pill/capsule format at frequent intervals daily to maintain a therapeutic dose of enzyme to achieve long-term clinical efficacy. This protocol will be the second oral ERT plant-made pharmaceutical (PMPs)(73,88-90,95). Thus, to provide a less expensive alternative, we generated a rhGAA produced in tobacco seeds for ERT of Pompe disease. We found that the tobrhGAA compared favorably and was superior on many aspects to alglucosidase alfa (Table 7). After scaling-up, we estimate that the yearly cost for an adults livng with Pompe disease would be $3,000 or <1% of the cost of alglucosidase alfa which ranges from $250,000 to $650,000 per adult patient per year depending upon weight. Successful demonstration of Oral-ERT with tobrhGAA will have significant clinical applications and shift current clinical practice paradigm by offering a safe, stable at room temperature and affordable lifelong Oral-ERT for Pompe disease in the USA and World-wide especially in areas where access to a clinical setting for biweekly IV administration of Myozyme is lacking. We estimate that an adults living with Pompe disease patient will need to take 3-4 grams daily at a cost of 0.1% to <1% of Myozyme depending on how it is branded and marketed to maintain a sustained GAA level of 5-10% of normal.
ACKNOWLEDGEMENTS
None
Competing interests: We have no declaration of interests and have no relevant interests to declare.
Research was supported in part by the NIH-SBIR Phase I grant-Oral-ERT of Pompe Disease with Tobacco Seed-Derived Recombinant Acid Maltase" RFA-AR-18-005 grant #1R43AR073522-01
Legends
Figure 1 Hydroponic growth of tobacco plants and processing of seeds Transgenic tobacco plant #3 expressing human GAA in seeds are grown indoors using a hydroponic system with deionized water (eliminating water/soil contaminants). Seed-pods are harvested and dried in freeze-dryer followed by separation from husk through a standard food mesh strainer. To eliminate environmental contamination, ground seeds were tested for lack of viability and germination. Seeds are grounded for 10 minutes in a porcelain pestle-mortar until a fine powder and placed in a UV germicidal incubator for 30 minutes to sterilize. Approximately, 0.1 gram of ground/sterilized seeds (~1,000 whole seeds) and untreated transgenic seeds are germinated (positive control) by evenly distributed in a petri dish on paper towels saturated with water and observed for 7-8 days at room temperature. Viable seeds will germinate in a week. No germination was observed in processed seeds
Figure 2 Long-term treatment and vertical hang-time measurements We treated 2 groups of GAA KO mice (exon 6neo)(n=3)-3x weekly with either a 1x or 3x dose (25 or 75 mg tobrhGAA/dose) orally and measured vertical hang-time over 203 days. Both treatment groups showed a steady significant improvement in vertical hang-time vs. mock treated KO mice. Interestingly, mice in the 1x group gradually increased hang-time equal to the 3x dose group, suggesting that the lower dose can be effective in long-term treatment. Both treatment groups showed 25-35% vs. mock treated wild-type mice at day 82, but by day 203, both groups were almost equal (89-95%) to mock treated wild-type mice.
Figure 3 Treatment with whole seeds and running wheel activity GAA KO mice (3 females- ~4 months old) were fed twice daily with 50 mg whole tobrhGAA seeds and running wheel activity monitored for 21 days, followed by 50 mg-1x daily for 14 days and then 50 mg every 2 days. Historical levels for wild-type and GAA KO mice and GAA KO mice feed 50 mg of wild-type tobacco seeds are indicted. GAA KO mice treated 2x daily with 50 mg tobrhGAA seeds showed increased running wheel activity to 60% of wild-type by day 24. At day 25, mice were feed 50 mg-1x daily, resulted in a reduction of RW to 40% of wild-type by day 40 and at day 41, mice were feed 50 mg every other day-reduced activity to mock treated levels.
Figure 4 Pharmacokinetics in serum of Oral-ERT in GAA KO mice Serum clearance rate with the 3x-dose (75 mg tobrhGAA) or whole seeds in GAA KO mice were collected at 0, 1, 2, 4, 6, 8, 10, 12, 24 and 48 hr. TobrhGAA activity showed maximum serum GAA concentration (Cs) at 8 to 10 hr.
Figure 5 Pharmacokinetics in urine of Oral-ERT in GAA KO mice Urine clearance rate with the 3x-dose (75 mg tobrhGAA) or whole seeds in GAA KO mice were collected at 0, 1, 2, 4, 6, 8, 10, 12, 24 and 48 hr. TobrhGAA activity showed maximum urine GAA excretion at 10 to 12 hr.
Graph 1 Uptake of tobrhGAA in human lymphoid cell lines Human lymphoid cell lines from infantile or adult Pompe disease (GM6314, GM13793, GM14450) were exposed to equivalent amounts of tobrhGAA, placental GAA or a rhGAA. Cells were harvested after 2, 24 and 48 hours and assayed for GAA and NAG. All treatments were significant at p = <0.05.
Graph 2 Uptake of tobrhGAA in human fibroblast cell lines Human fibroblast (GM4912, GM1935, GM3329) cell lines from infantile or adult Pompe disease were exposed to equivalent amounts of tobrhGAA, placental GAA or a rhGAA. Cells were harvested after 2, 24 and 48 hours and assayed for GAA and NAG. All treatments were significant at p = <0.05.
Graph 3 Uptake of tobrhGAA in a human Pompe disease myoblast cell line A human Pompr disease myoblast cell line was exposed to equivalent amounts of a lysate of tobrhGAA or an rhGAA for 48 hr and assayed for GAA and NAG. We found that tobrhGAA increased GAA to 24-35% of normal (mean+SD).
Graph 4 Taken up by a distant tissue We determined if the tobrhGAA was taken up by a distant tissue-tail without sacrificing the mice and assayed for GAA at 45 and 203 days. Wild-type mice had GAA activity of 0.46+0.14 (SD), mock treated GAA KO had 0.064+0.021 and treated GAA KO had 0.10 to 0.2+0.05 for 1x or 3x dose (p = <0.006 treated vs mock treated).
Graph 5 Assessment of spontaneous alternation in wild-type and GAA KO mice We assessed spontaneous alternative learning for cognitive ability in the T-maze in both male and female GAA KO mice and WT-129/C57 mice from 2-9 months of age. We found that deficiency in spontaneous learning appeared by 2-3 months in male and 3-4 months in female GAA KO mice. All conditions were significant (p = <0.05).
Figures, Tables and Graphs
Fig. 1 Unground seeds
day 7 day 8 day 10
Ground and UV treated seeds
day 0 day 8 day 10
Table 1
Enzyme kinetics
*from literature for
various species and tissue sources
Human mature placental GAA
tobrhGAA
Myozyme**or other rhGAAs** published data for Myozyme is limited
Substrate
Km*
Vmax*
Km Vmax
Km Vmax
Km Vmax
glycogen
10-42 mg/ml
6.63 mmole glucose/mg/hr
18mg/ml 5.2
21mg/ml 5.8
maltose
2-22 mM
14.3 U/mg
16 mM 0.186 mmol/mg/hr
4-MU-Glyc
0.8-1.1mM
0.55mmole/mg/hr
0.95 mM 0.85
0.85 mM 0.65
1.08-2.1 mM 0.07-0.548 mmole/mg/hr
isomaltose
40mM
To be done
To be done
starch
15.4mM
10 U/mg
To be done
To be done
Inhibitors IC50
Acarbose on 4-MU-Glc
on glycogen
81 umol/L
54 umole/L
85 umol/L
75 umol/L
59 umol/L
Maltose on 4-MU-Glc
45 mM
45 mM
?
Glycogen on 4-MU-Glc
15 mg/ml
10 mg/ml
?
pH optima
4-5.5
4-4.5 for 4-MU-Glc
4-4.5 for 4-MU-Glc
4-4.25 for glycogen 4-5.5 for 4-MU-Glc
Heat Stability
Variable depending on methods used.
<5% residual activity after 5 min at 56oC
<5% residual activity after 5 min at 56oC
<5% residual activity after 24 hrs at 37oC
Table 2. GAA/NAG (mean ± SD) assay of mouse tissues after administration of tobrhGAA for 3x over 7 days via oral gavage.
Hind-limb tibialis anterior Heart Diaphragm Liver Skeletal Muscle
Treated GAA KO - Oral tobrhGAA 0.12 ± 0.04 0.10 ± 0.06 0.13 ± 0.09 0.19 ± 0.02
Mock GAA KO- PBS 0.043 ± 0.006 0.06 ± 0.01 0.07 ± 0.03 0.05 ± 0.009
Wild-type-Balb/c 1.50 ± 0.2 0.43 ± 0.19 0.77 ± 0.15 1.00 ± 0.13 % of WT 8% 23% 17% 19%
TABLE 3 GAA/NAG (mean ± SD) assay of mouse tissues after administration of tobrhGAA via oral gavage (Oral-ERT) at day 203.
Hind-limb tibialis anterior Heart Diaphragm Liver Kidney Skeletal Muscle
Treated GAA KO –1x Oral tobrhGAA 0.11 ± 0.04 0.14 ± 0.02 0.24 ± 0.01 0.17 ± 0.03 0.24 ± 0.04
% of WT 14% 18% 37% 13% 22%
Treated GAA KO –3x Oral tobrhGAA 0.13 ± 0.01 0.13 ± 0.01 0.25 ± 0.01 0.21 ± 0.05 0.23 ± 0.01
% of WT 16% 17% 38% 16% 20%
Mock GAA KO- PBS 0.07 ± 0.044 0.065 ± 0.044 0.08 ± 0.14 0.05 ± 0.009 1.35 ± 0.02
Wild-type 0.8 ± 0.11 0.76 ± 0.23 0.65 ± 0.38 1.33 ± 0.36 1.12 ± 0.08 TABLE 4 % glycogen reduction (mean) in mouse tissues after administration of tobrhGAA at day 203 (Oral-ERT).
Hind-limb tibialis anterior Heart Diaphragm Liver Kidney
Skeletal Muscle
Treated GAA KO -1x Oral tobrhGAA 39 32 48 18 28
Treated GAA KO -3x Oral tobrhGAA 43 18 24 25 29
Table 5
RW
GSM
Rotarod
T-maze
Open-field mobility
CBC diff.
Mean+SD
rev/12 hrs
lbs at release
Time (sec)
% correct alternation
cm/min +SD
Pre-Tx n = 7-37 M/F
404+128
0.80+59
25+49
32+25
182+79
GAA KO M/F-50mg n = 4
3 weeks
1800+80*
0.310+133*
158+138*
82+5*
243+46
Nl.
6 weeks
2528+60*
0.394+178*
171+70*
65+9*
449+53*
Nl.
12 weeks
2194+57*
0.304+126*
258+103*
70+2*
436+63*
Nl
GAA KO M/F-150mg n = 3
3 weeks
436+40
0.307+138*
120+73*
86+1*
442+75*
Nl.
6 weeks
1248+55*
0.452+147*
323+57*
81+3*
432+49*
Nl.
12 weeks
2549+68*
0.397+180*
355+104*
67+15*
344+54*
Nl
WT
M/F n = 12-15
3,200+1423
0.569+296
264+101
60+16
334+96
*p=<0.05 when compared to pre-treatment data by T-test (1-tail, 2-equal variance)
TABLE 6
liver
heart
Hind-limb tibialis anterior muscle
kidney
lung
diaphragm
brain
SI-D
SI-J
SI-I
spleen
pancreas
GAA KO mock
0.073
0.06
0.018
0.085
0.094
0.045
0.012
0.4
0.94
0.71
0.015
0.084
GAA KO Tx 50mg
0.54
0.1
0.053
0.3
0.15
0.11
0.023
1.57
1.83
1.54
0.022
0.14
GAA KO Tx 150mg
0.64
0.2
0.08
0.78
0.3
0.2
0.05
2
6
5.1
0.07
0.45
WT-129/C57
4.3
3.1
0.71
1.67
0.31
0.37
0.73
2.68
3.47
3.3
1.06
0.67
% WT 50/150mg
13/15
3/6
7/11
18/47
50/100
30/54
3/7
58/75
53/173
47/154
2/7
21/67
Table 7
Issues with the Current Therapy-Myozyme Our Oral-ERT with tobrhGAA addresses these issues
> Inefficient uptake Efficient uptake in appropriate tissues
> Transient nature-once every 2 weeks Daily Oral-ERT
> Glycogen reduction is variable Glycogen reduction
> Autophagic and lipofuscin accumulation to be determined
> Immune response Immune response minimized
> High cost Projected lower cost of plant made pharmaceuticals (PMPs) (1-10% of current therapy depending upon level of purification needed)
> Residual effective level Sustained daily increased residual GAA level
> Requires refrigeration and a clinic visit Extremely stable at room temperature for many years and can be administer ERTprovided Worldwide without refrigeration
REFERENCES
1.Slonim A, Bulone L, Ritz S, Goldberg T, Chen A, Martiniuk F. Identification of two subtypes of infantile acid maltase deficiency: Evaluation of twenty-two patients and review of the literature. J Pediatrics. 2000;137:283-5.
2.Engel AG. Acid maltase deficiency. In Engel AG, Franzini-Armstrong C, eds. Myology, N.Y., McGraw Hill, Inc. p. 1533-1553, 1994.
3.Reuser AJJ, Kroos M, Willemsen R, Swallow D, Tager JM, Galjaard H. Clinical diversity in glycogenosis type II. J Clin Invest. 1987;79:1689-1699.
4.Beratis N, LaBadie GU, Hirschhorn R. Genetic heterogeneity in acid alpha glucosidase deficiency. Am J Hum Genet. 1983;35:21-33.
5.Tagers JM, Oude Elferink RPJ, Reuser AJJ, Kroos M, Ginsel LA, Franzen JAM, Klumperman J. Alpha glucosidase deficiency; Pompe's disease. Enzyme. 1987;38:280-285.
6.van den Hout HM, Hop W, van Diggelen OP, Smeitink JA, Smit GP, Poll-The BT, Bakker HD, Loonen MC, de Klerk JB, Reuser AJ, van der Ploeg AT. The natural course of infantile Pompe's disease: 20 original cases compared with 133 cases from the literature. Pediatrics. 2003;112:332-340.
7.Winkel LP, Hagemans ML, van Doorn PA, Loonen MC, Hop WJ, Reuser AJ, van der Ploeg AT. The natural course of non-classic Pompe's disease; a review of 225 published cases. J Neurol. 2005;252:875-884.
8.Engel AG, Gomez MR, Seybold ME, Lambert EH. The spectrum and diagnosis of acid maltase deficiency. Neurology. 1973;23:95-106.
9.Mehler M, DiMauro S. Residual GAA activity in late-onset acid maltase deficiency. Neurol. 1997;27:178-184.
10.La Badie GU. Biochemical and immunologic studies of acid alpha glucosidase deficiency, a genetically heterogeneous, inherited neuromuscular disease. (Ph.D. Thesis, City University of New York, Mt. Sinai Hospital, 1986).
11.Raben N, Ralston E, Chien YH, Baum R, Schreiner C, Hwu WL, Zaal KJ, Plotz PH. Differences in the predominance of lysosomal and autophagic pathologies between infants and adults with Pompe disease: implications for therapy. Mol Genet Metab. 2010;101:324-331.
12.Martiniuk F, Chen A, Mack A, Codd W, Hanna B, Arvanitopoulos E, Slonin A. Carrier frequency for glycogen storage disease type II in New York and estimates of affected individuals born with the disease. Am J Med Genet. 1998;79:69-72.
13.Park K.S. Carrier frequency and predicted genetic prevalence of Pompe disease based on a general population database. Molecular Genetics and Metabolism Reports. 2021;27:100734-42.
14.Reuser AJJ, Kroos M, Elferink RPJO, Tager JM. Defects in synthesis, phosphorylation, and maturation of acid alpha glucosidase. J Biol Chem. 1985;260:8336-8341.
15.Strothotte S, Strigl-Pill N, Grunert B, Kornblum C, Eger K, Wessig C, Deschauer M, Breunig F, Glocker FX, Vielhaber S, Brejova A, Hilz M, Reiners K, Müller-Felber W, Mengel E, Spranger M, Schoser B. Enzyme replacement therapy with alglucosidase alfa in 44 patients with late-onset glycogen storage disease type 2:12-month results of an observational clinical trial. J Neurol. 2010;257:91-97.
16.van der Ploeg AT, Clemens PR, Corzo D, Escolar DM, Florence J, Groeneveld GJ, Herson S, Kishnani PS, Laforet P, Lake SL, Lange DJ, Leshner RT, Mayhew JE,Morgan C, Nozaki K, Park DJ, Pestronk A, Rosenbloom B, Skrinar A, van Capelle CI, van der Beek NA, Wasserstein M, Zivkovic SA. A randomized study of alglucosidase alfa in late-onset Pompe's disease. N Engl J Med. 2010;362:1396-1406.
17.Douillard-Guilloux G, Raben N, Takikita S, Ferry A, Vignaud A, Guillet-Deniau I, Favier M, Thurberg BL, Roach PJ, Caillaud C, Richard E. Restoration of muscle functionality by genetic suppression of glycogen synthesis in a murine model of Pompe disease. Human Molecular Genetics. 2010;19:684–696.
18.Lim J, Li L, Raben N. Pompe disease: from pathophysiology to therapy and back again. Frontiers in Aging Neuroscience. 2014;6:1-14.
19.van Gelder CM, Hoogeveen-Westerveld M, Kroos MA, Plug I, van der Ploeg AT, Reuser AJJ. Enzyme therapy and immune response in relation to CRIM status: the Dutch experience in classic infantile Pompe disease. J Inherit Metab Dis. 2015;38:305–314.
20.Lacana´ E, Yao LP, Pariser AR, Rosenberg AS. The role of immune tolerance induction in restoration of the efficacy of ERT in Pompe disease. Am J Med Genet Part C Semin Med Genet. 2012;160C:30–39.
21.Bali DS, Goldstein JL, Banugaria S, Dai J, Mackey J, Rehder C, Kishnani PS. Predicting cross-reactive immunological material (CRIM) status in Pompe disease using GAA mutations: Lessons learned from 10 years of clinical laboratory testing experience. Am J Med Genet Part C Semin. 2012;160C:40–49.
22.Joseph A, Munroe K, Housman M, Garman R, Richards S. Immune tolerance induction to enzyme-replacement therapy by co-administration of short-term, low-dose methotrexate in a murine Pompe disease model. Clinical and Experimental Immunology. 2013;152:138–146.
23.Banugaria SG, Prater SN, McGann JK, Feldman JD, Tannenbaum JA, Bailey C, Gera R, Conway RL, Viskochil D, Kobori JA, Rosenberg AS, Kishnani PS. Bortezomib in the rapid reduction of high sustained antibody titers in disorders treated with therapeutic protein: lessons learned from Pompe disease. Genet Med. 2013;15:123–131.
24.Berrier KL, Kazi ZB, Prater SN, Bali DS, Goldstein J, Stefanescu MC, Rehder CW, Botha EG, Ellaway C, Bhattacharya K, Tylki-Szymanska A, Karabul N, Rosenburg AS, Kishnani PS. CRIM-negative infantile Pompe disease: characterization of immune responses in patients treated with ERT monotherapy. Genet Med. 2015;17:912-918.
25.Banugaria SG, Prater SN, Ng YK, Kobori JA, Finkel RS, Ladda RL, Chen YT, Rosenberg AS, Kishnani PS. The impact of antibodies on clinical outcomes in diseases treated with therapeutic protein: Lessons learned from infantile Pompe disease. Genetics in Medicine. 2011;13:729-736.
26.Messinger YH, Mendelsohn NJ, Rhead W, Dimmock D, Hershkovitz E, Champion M, Jones SA, Olson R, White A, Wells C, Bali D, Case LE, Young SP, Rosenberg AS, Kishnani PS. Successful immune tolerance induction to enzyme replacement therapy in CRIM–negative infantile Pompe disease. Genet Med. 2012:14:135–142.
27.Al Khallaf HH, Geffrard J, Botha E, Ali Pervaiz M. CRIM-negative Pompe disease patients with satisfactory clinical outcomes on enzyme replacement therapy. JIMD Reports. 2012;192:133-7.
28.Brooks DS. Immune response to enzyme replacement therapy in lysosomal storage disorder patients and animal models. Molecular Genetics and Metabolism. 1999;68:268–275.
29.Kishnani PS, Goldenberg PC, DeArmey SL, Heller J, Benjamin D, Young S, Bali D, Smith SA, Li JS, Mandel H, Koeberl D, Rosenberg A, Chen YT. Cross-reactive immunologic material status affects treatment outcomes in Pompe disease infants. Molecular Genetics and Metabolism. 2010;99:26–33.
30.de Vries JM, van der Beek N, Kroos MA, Özkan L, van Doorn PA, Richards SM, Sung CCC, Brugma JDC, Zandbergen AAM, van der Ploeg AT, Reuser AJJ. High antibody titer in an adult with Pompe disease affects treatment with alglucosidase alfa. Molecular Genetics and Metabolism. 2010;101:338–345.
31.Patel TT, Banugaria SG, Case LE, Wenninger S, Schoser B, Kishnani PS. The impact of antibodies in late-onset Pompe disease: A case series and literature review. Molecular Genetics and Metabolism. 2012;106:301–309.
32.Wang J, Lozier J, Johnson G, Kirshner S, Verthelyi D, Pariser A, Shores E, Rosenberg A. Neutralizing antibodies to therapeutic enzymes: considerations for testing, prevention and treatment. Nature Biotechnology. 2008;26:901-08.
33.Mendelsohn NJ, Messinger YH, Rosenberg AS, Kishnani PS. Elimination of antibodies to recombinant enzyme in Pompe’s disease. NEJM. 2009;360;194-5.
34.Hunley TE, Corzo D, Dudek M, Kishnani P, Amalfitano A, Chen YT, Richards SM, Phillips JA, Fogo AB, Tiller GE. Nephrotic syndrome complicating a-glucosidase replacement therapy for Pompe disease. Ped. 2004;114:e5325.
35.Banugaria SG, Prater N, Patel TT, DeArmey SM, Milleson C, Sheets KB, Bali DS, Rehder CW, Raiman JA, Wang RA, Labarthe F, Charrow J, Harmatz P, Chakraborty P, Rosenberg AS, Kishnani PS. Algorithm for the early diagnosis and treatment of patients with cross reactive immunologic material-negative classic Infantile Pompe disease: A step towards improving the efficacy of ERT. PLoS ONE. 2013;8:e67052-12.
36.Nayak S, Doerfler PA, Porvasnik SL, Cloutier DD, Khanna R, Valenzano KJ, Herzog RW, Byrne BJ. Immune responses and hypercoagulation in ERT for Pompe disease are mutation and rhGAA dose dependent. PLoS ONE. 2014;9:e98336-47.
37.de Vries JM, Kuperus E, Hoogeveen-Westerveld M, Kroos MA, Wens CAA, Stok M, van der Beek NAME, Kruijshaar ME, Rizopoulos D, van Doorn PA, van der Ploeg AT, Pim Pijnappel WWM. Pompe disease in adulthood: effects of antibody formation on enzyme replacement therapy. Genet Med. 2016;19:90-97.
38.Ohashi T, Iizuka S, Shimada Y, Eto Y, Ida H, Hachimura S, Kobayashi H. Oral administration of recombinant human acid α-glucosidase reduces specific antibody formation against enzyme in mouse. Molecular Genetics and Metabolism. 2011;103:98–100.
39.Parini R, De Lorenzo P, Dardis A, Burlina A, Cassio A, Cavarzere P, Concolino D, Della Casa R, Deodato F, Donati MA, Fiumara A, Gasperini S, Menni F, Pagliardini V, Sacchini M, Spada M, Taurisano R, Grazia Valsecchi M, Di Rocco M, Bembi B. Long term clinical history of an Italian cohort of infantile onset Pompe disease treated with enzyme replacement therapy. Orphanet Journal of Rare Diseases. 2018;13:32-44.
40.Nayak S, Doerfler PA, Porvasnik SL, Cloutier DD, Khanna R, Valenzano KJ, Herzog RW, Byrne BJ. Immune responses and hypercoagulation in ERT for Pompe disease are mutation and rhGAA dose dependent. PLoS ONE. 2014;9:e98336-47.
41.de Vries, J.M., Kuperus, E., Hoogeveen-Westerveld, M., Kroos, M.A., Wens, S.C.A., Stok, M., van der Beek, N.A.M.E., Kruijshaar, M.E., Rizopoulos, D., van Doorn, P.A., van der Ploeg, A.T. and Pim Pijnappel, W.W.M. Pompe disease in adulthood: effects of antibody formation on enzyme replacement therapy. Genetics in Medicine. 2017;19:90-97.
42.Gutschmidt K, Musumeci O, Díaz-Manera J, Chien Y-H, Knop KC, Wenninger S, Montagnese F, Pugliese A, Tavilla G, Alonso-Pérez J, Wuh-Liang Hwu P, Toscano A, Schoser B. STIG study: real-world data of long-term outcomes of adults with Pompe disease under enzyme replacement therapy with alglucosidase alfa. J Neurol. 2021; 5:1–11.
43.Raben N, Jatkar T, Lee A, Lu N, Dwivedi S, Nagaraju K, Plotz PH. Glycogen stored in skeletal but not in cardiac muscle in acid a-glucosidase mutant (Pompe) mice is highly resistant to transgene-encoded human enzyme. Molecular Therapy. 2002;6:601-608.
44.Neufeld EF. Lysosomal storage diseases. Annu Rev Biochem. 1991;60:257–280.
45.Van den Hout JM, Reuser AJ, de Klerk JB, Arts WF, Smeitink JA, Van der Ploeg AT. Enzyme therapy for Pompe disease with recombinant human a-glucosidase from rabbit milk. J Inherit Metab Dis. 2001;24:266–274.
46.Reuser AJ, Kroos MA, Hermans MM, Bijvoet AG, Verbeet MP, Van Diggelen OP, Kleijer WJ, van der Ploeg AT. Glycogenosis type II (acid maltase deficiency). Muscle Nerve. 1995;3S:61–69.
47.van den Hout H, Reuser AJ, Vulto AG, Loonen MC, Cromme-Dijkhuis A, van der Ploeg AT. Recombinant human a-glucosidase from rabbit milk in Pompe patients. Lancet. 2000;356:397–398.
48.Amalfitano A, Bengur AR, Morse RP, Majure JM, Case LE, Veerling DL, Mackey J, Kishnani P, Smith W, McVie-Wylie A, Sullivan JA, Hoganson GE, Phillips JA 3rd, Schaefer GB, Charrow J, Ware RE, Bossen EH, Chen YT. Recombinant human acid a-glucosidase enzyme therapy for infantile glycogen storage disease type II: results of a phase I/II clinical trial. Genet Med. 2001;3:132–138.
49.Corti M, Liberati C, Smith BK, Lawson LA, Tuna IS, Conlon TJ, Coleman KE, Islam S, Herzog RW, Fuller DD, Collins SW, Byrne BJ. Safety of intradiaphragmatic delivery of adeno-associated virusmediated alpha-glucosidase (rAAV1-CMV-hGAA) gene therapy in children affected by Pompe disease. Hum Gene Ther Clin. Dev. 2017;28:208–1.
50.Miyamoto Y, Etoh Y, Joh R, Noda K, Ohya I. Morimatsu M. Adult-onset acid maltase deficiency in siblings. Acta Pathol. Jpn. 1985;35:1533–42.
51.Anneser JMH, Pongratz DE, Podskarbi T, Shin YS, Schoser BGH. Mutations in the acid α-glucosidase gene (M. Pompe) in a patient with an unusual phenotype. Neurology 2005;64:368–70.
52.Refai D, Lev R, Cross DT, Shimony JS, Leonard JR. Thrombotic complications of a basilar artery aneurysm in a young adult with Pompe disease. Surg Neurol. 2008;70:518–20.
53.Kretzschmar HA, Wagner H, Hubner G, Danek A, Witt TN, Mehraein P. Aneurysms and vacuolar degeneration of cerebral arteries in late-onset acid maltase deficiency. J Neurol Sci. 1990;98:169–83.
54.Makos MM, McComb RD, Hart MN, Bennett DR. Alpha-glucosidase deficiency and basilar artery aneurysm: report of a sibship. Ann Neurol. 1987;22:629–33.
55.Kobayashi H, Shimada Y, Ikegami M, Kawai T, Sakurai K, Urashima T, Ijima M, Fujiwara M, Kaneshiro E, Ohashi T, Eto Y, Ishigaki K, Osawa M, Kyosen SO, Ida H. Prognostic factors for the late onset Pompe disease with enzyme replacement therapy: from our experience of 4 cases including an autopsy case. Mol Genet Metab. 2010;100:14–9.
56.McCall AL, Dhindsa JS, Bailey AM, Pucci LA, Strickland LM, Eimallah MK. Glycogen accumulation in smooth muscle of a Pompe disease mouse model. J Smooth Muscle Res. 2021;57:8–18.
57.Hundsberger T, Rohrbach M, Kern L, Rösler KM. Swiss national guideline for reimbursement of enzyme replacement therapy in late-onset Pompe disease. J Neurol. 2013;260:2279-85.
58.Kusnadi AR, Evangelista RL, Hood EE, Howard JA, Nikolov ZL. Processing of transgenic corn seed and its effect on the recovery of recombinant beta-glucuronidase. Biotechnol Bioeng. 1998;60:44–52.
59.Reggi S, Marchetti S, Patti T, De Amicis F, Cariati R, Bembi B, Fogher C. Recombinant human acid b-glucosidase stored in tobacco seed is stable, active and taken up by human fibroblasts. Plant Molecular Biology. 2005;57:101–113.
60.Stoeger E, Vaquero C, Torres E, Sack M, Nicholson L, Drossard J, Williams S, Keen D, Perrin Y, Christou P, Fischer R. Cereal crops as viable production and storage systems for pharmaceutical scFv antibodies. Plant Mol Biol. 2000;42:583–590.
61.Kermode AR. Plants as factories for production of biopharmaceutical and bioindustrial proteins: lessons from cell biology. Can J Bot. 2006;84,679–694.
62.Kermode AR. Seed Expression Systems for Molecular Farming. In: Wang, A., Ma, S. (eds) Molecular farming in plants: recent advances and future prospects. Springer, New York, 2012;89–123.
63.Lau OS, Sun SSM. Plant seeds as bioreactors for recombinant protein production. Biotechnol Adv. 2009;27:1015–1022.
64.Fischer R, Schillberg S, Hellwig S, Twyman RM, Drossard J. GMP issues for recombinant plant-derived pharmaceutical proteins. Biotechnol Adv. 2012;30:434-439.
65.Lico C, Santi L, Twyman RM, Pezzotti M, Avesani L. The use of plants for the production of therapeutic human peptides. Plant Cell Rep. 2012;31:439-451.
66.Twyman RM, Ramessar K, Quemada H, Capell T, Christou P. Plant biotechnology: the importance of being accurate. Trends Biotechnol. 2009;27:609-612.
67.Twyman RM, Stoger E, Schillberg S, Christou P, Fischer R. Molecular farming in plants: host systems and expression technology. Trends Biotechnol. 2003;21:570-578.
68.Kwon K, Verma D, Singh N, Herzog R, Daniell H. Oral delivery of human biopharmaceuticals, autoantigens and vaccine antigens bioencapsulated in plant cells. Adv Drug Deliv Rev. 2013;65:782–799.
69.Verma D, Moghimi B, LoDuca PA, Singh H, Hoffman BE, Herzog R, Daniell H. Oral delivery of bioencapsulated coagulation factor IX prevents inhibitor formation and fatal anaphylaxis in hemophilia B mice. PNAS. 2010;107:7101–7106.
70.Sherman A, Su J, Lin S, Wang X, Herzog RW, Daniell H. Suppression of inhibitor formation against FVIII in a murine model of hemophilia A by oral delivery of antigens bioencapsulated in plant cells. Blood. 2014;124:1659-68.
71.Boothe J, Nykiforuk C, Shen Y, Zaplachinski S, Szarka S, Kuhlman P, Murray E, Morck D, Moloney MM. Seed-based expression system for plant molecular farming. Plant Biotechnol J. 2010;8:588–606.
72.Kwon K-C, Daniell H. Low-cost oral delivery of protein drugs bioencapsulated in plant cells. Plant Biotechnol J. 2015;13:1017–1022.
73.Kaufman J. The economic potential of plant-made pharmaceuticals in the manufacture of biologic pharmaceuticals. J Commercial Biotechnology. 2011;17:173-182.
74.Kaiser J. Is the drought over for Pharming? Science. 2008;320:473-475.
75.Maxmen A. Drug making plant blooms. Nature. 2012;485:160.
76.Spok A, Karner S. Plant made farming-opportunities and challenges. JRC European Comm.
2008;JJRC43873:1-148.
77.Lerouge P, Cabanes-Macheteau M, Rayon C, Fischette-Lainé AC, Gomord V, Faye L. N-glycosylation of recombinant pharmaceutical glycoproteins produced in transgenic plants: towards an humanisation of plant N-glycans. Plant Mol Biol. 1998;38:31-48.
78.Boothe J, Nykiforuk C, Shen Y, Zaplachinski S, Szarka S, Kuhlman P, Murray E, Morck D, Moloney MM. Seed-based expression system for plant molecular farming. Plant Biotechnol J. 2010;8:588-606.
79.Stoeger E, Ma JK. Sowing the seeds of success: Pharmaceutical proteins from plants. Curr Opin Biotechnol. 2005;16:167-173.
80.Kusnadi AR, Evangelista RL, Hood EE, Howard JA, Nikolov ZL. Processing of transgenic corn seed and its effect on the recovery of recombinant beta-glucuronidase. Biotechnol Bioeng. 1998;60:44-52.
81.Gomord V, Fischette AC, Menu-Bouaouiche L, Saint-Jore-Dupas C, Plasson C, Michaud D, Faye L. Plant-specific glycosylation patterns in the context of therapeutic protein production. Plant Biotechnol J. 2010;8:564-587.
82.Saint-Jore-Dupas C, Faye L, Gomord V. From planta to pharma with glycosylation in the toolbox. Trends Biotechnol. 2007;25:317-323.
83.Gomord V, Faye L. Posttranslational modification of therapeutic proteins in plants. Curr Opin Plant Biol. 2004;7:171-181.
84.Kermode AR. Mechanisms of intracellular protein transport and targeting. Crit Rev Plant Sci. 1996;15:285-423.
85.He X, Galpin JD, Tropak MB, Mahuran D, Haselhorst T, von Itzstein M, Kolarich D, Packer NH, Miao Y, Jiang L, Grabowski GA, Clarke LA, Kermode AR. Production of active human glucocerebrosidase in seeds of Arabidopsis thaliana complex-glycan-deficient (cgl) plants. Glycobiology. 2012;22:492-503.
86.Aviezer D, Almon-Brilla E,Y, Chertkoffa R, Hashmuelia S, Zimranb A. Novel enzyme replacement therapy for Gaucher disease: Phase III pivotal clinical trial with plant cell expressed recombinant glucocerebrosidase (prGCD)–taliglucerase alpha. Mol Gen Metab. 2010;99:S9.
87.Shaaltiel Y, Bartfeld D, Hashmueli S, Baum G, Brill-Almon E, Galili Orly Dym G, Boldin-Adamsky SA, Silman I, Sussman JL, Futerman AH, Aviezer D. Production of glucocerebrosidase with terminal mannose glycans for enzyme replacement therapy of Gaucher’s disease using a plant cell system. Plant Biotechnology J. 2007;5:579–590.
88.Grabowski GA, Golembo M, Shaaltiel Y. Taliglucerase alfa: an enzyme replacement therapy using plant cell expression technology. Mol Gen Met. 2014;112:1-8.
89.Tekoah Y, Shulman A, Kizhner T, Ruderfer I, Fux L, Nataf Y, Bartfeld D, Ariel T, Gingis–Velitski S, Hanania U, Shaaltiel Y. Large-scale production of pharmaceutical proteins in plant cell culture—the Protalix experience. Plant Biotechnology J. 2015;13:1199-1208.
90.Shaaltiel Y, Gingis–Velitski S, Tzaban S, Fiks N, Tekoah Y, Aviezer D. Plant-based oral delivery of b-glucocerebrosidase as an enzyme replacement therapy for Gaucher’s disease. Plant Biotechnology J. 2015;13:1033-1040.
91.Martiniuk F, Reggi S, Tchou-Wong K-M, Rom WN, Busconi M, Fogher C. Production of a functional human acid maltase in tobacco seeds: biochemical analysis, uptake by human GSDII cells, and in vivo studies in GAA knockout mice. Appl Biochem Biotechnol. 2013;171:916-926.
92.Martiniuk F, Chen A, Donnabella V, Arvanitopoulos E, Slonim AE, Raben N, Plotz P, Rom WN. Correction of glycogen storage disease type II by enzyme replacement with a recombinant human acid maltase produced by over-expression in a CHO-DHFR (neg) cell line. Biochem Biophys Res Commun. 2000;276:917-923.
93.Horsch RB, Fry JE, Hoffmann NL, Eichholtz D, Roger SD, Fraley RT. A simple 344 and general method for transferring genes into plants. Science. 1985;227:1229-1231.
94.Martiniuk F, Honig J, Hirschhorn R. Further studies of the structure of human placental acid alpha-glucosidase. Arch Biochem Biophys. 1984;231:454-460.
95.Paul M, Ma J. Plant-made pharmaceuticals:leading products and production. Biotech Applied Biochem. 2011;58:58-67.
96.Dressman JB, Amidon GL, Reppas C, Shah VP. Dissolution testing as a prognostic tool for oral drug absorption:immediate release dosage forms. Pharm Res. 1998;15:11-22.
97.Martiniuk F, Hirschhorn, R. Characterization of neutral isozymes of human alpha-glucosidase. Biochim Biophys Acta. 1981;658:248-261.
98.Kakkis E, Lester T, Yang R, Tanaka C, Anand V, Lemontt J, Peinovich M, Passage M. Successful
induction of immune tolerance to enzyme replacement therapy in canine mucopolysaccharidosis I. Proc Natl Acad Sci. 2004;101:829-834.
99.White RR , Crawley FE, Vellini M, Rovati LA. Bioavailability of 125I bromelain after oral administration to rats. Biopharm Drug Dispos. 1988;9:397-403.
100.Van Hove JK, Yang HW, Wu J-Y, Brady RO, Chen YT. High-level production of recombinant human lysosomal acid a-glucosidase in Chinese hamster ovary cells which targets to heart muscle and corrects glycogen accumulation in fibroblasts from patients with Pompe disease. Proc Natl Acad Sci. 1996;93:65-70.
101.Fuller M, Van der Ploeg A, Reuser AJJ, Anson DS, Hopwood JJ. Isolation and characterisation of a recombinant, precursor form of lysosomal acid a-glucosidase. Eur J Biochem. 1995;234:903-900.
102.Hirschhorn R, Reuser AJJ. Glycogen storage disease type 2 acid alpha-glucosidase acid maltase deficiency. Macgraw-Hill, Chapter 135, 2001;3389-3420.
103.Chadalavada DM, Sivakami S. Purification and biochemical characterisation of human placental acid a-glucosidase. Biochemistry and Molecular Biology International. 1997;42:1051-1061.
104.Bijvoet AGA, Kroos MA, Pieper FR, Van der Vliet M, De Boer HA, van der Ploeg AT, Verbeet M, Reuser AJJ. Recombinant human acid a-glucosidase: high level production in mouse milk, biochemical characteristics, correction of enzyme deficiency in GSDII KO mice. Human Molecular Genetics. 1998;7:1815–1824.
105.van Diggelen OP, Oemardien LF, van der Beek NAME, Kroos MA, Wind HK, Voznyi YV, Burke D, Jackson M, Winchester BG, Reuser AJJ. Enzyme analysis for Pompe disease in leukocytes; superior results with natural substrate compared with artificial substrates. J Inherit Metab Dis. 2009;32:416–423.
106.Okumiya T, Keulemans JLM, Kroos MA, van der Beek NME, Boer MA, Takeuchi H, Van Diggelen OP, Reuser AJJ. A new diagnostic assay for glycogen storage disease type II in mixed leukocytes. Molecular Genetics and Metabolism. 2006;88:22–28.
107.Porto C, Ferrara MC, Meli M, Acampora E, Avolio V, Rosa M, Cobucci-Ponzano B, Colombo G, Moracci M, Andria G, Parenti G. Pharmacological enhancement of α-glucosidase by the allosteric chaperone N-acetylcysteine. Molecular Therapy. 2012;20:2201–2211.
108.Khanna R, Flanagan JJ, Feng J, Soska R, Frascella M, Pellegrino LJ, Lun Y, Guillen D, Lockhart DJ, Valenzano KJ. The pharmacological chaperone AT2220 increases recombinant human acid a-glucosidase uptake and glycogen reduction in a mouse model of Pompe disease. PLoS ONE. 2012;7:e40776.
109. Hintze S, Limmer S, Dabrowska-Schlepp P, Berg B, Krieghoff N, Busch A, Schaaf A, Meinke P, Schoser B. Moss-derived human recombinant GAA provides an optimized enzyme uptake in differentiated human muscle cells of Pompe disease. Int J Mol Sci. 2020;21:2642-2657.
110. Raben N, Nagaraju K, Lee E, Kessler P, Byrne B, Lee L, LaMaurex M, King J, Sauer B, Plotz P. Targeted disruption of the acid alpha glucosidase gene in mice causes an illness with critical features of both infantile and adult human glycogen storage disease type II. JBC. 1998;273:19086-92.
111.Wheeler TL, Eppolito AK, Smith LN, Huff TB, Smith RF. A novel method for oral stimulant administration in the neonate rat and similar species. J Neuroscience Methods. 2007;159:282–285.
112.Martiniuk F, Chen A, Mack A, Donnabella V, Slonim A, Bulone L, Arvanitopoulos E, Raben N, Plotz P, Rom WN. Helios gene gun particle delivery for therapy of acid maltase deficiency. DNA and Cell Biol. 2002;21:717-725.
113.Ames BN, Lee FD, Durston WE. An improved bacterial test system for the detection and classification of mutagens and carcinogens. Proc Nat Acad Sci USA. 1973;70:782-786.
114.Gomez-Lechon MJ, Ponsoda X, Castell JV. A microassay for measuring glycogen in 96-well-cultured cells. Analytical Biochemistry. 1996;236:296–301.
115.Lin K, Wang A. UV mutagenesis in Escherichia coli K-12: Cell survival and mutation frequency of the chromosomal genes lacZ, rpoB, ompF, and ampA. J Experimental Microbiology and Immunology. 2001;1:32-46.
116.Reesora MJ, King IJ, Michael J. Development of a tetracycline resistant strain of E. coli sensitive to ultraviolet radiation. J Student Research. 2014;3:63-68.
117.Liu A, Tran L, Becket E, Lee K, Chinn L, Park E, Tran K, Miller JH. Antibiotic sensitivity profiles determined with an Escherichia coli gene knockout collection: generating an antibiotic bar code. Antimicro Agents Chemo. 2010;54:1393-1403.
118.National Toxicology Program. The Salmonella/E. coli mutagenicity. http://ntp.niehs.nih.gov/go/9407. 2014;June 27.
119.Cheung SCK, Liu L, Lan L, Liu Q, Sun SSM, Chan JCN, Tong PCY. Glucose lowering effect of transgenic human insulin-like growth factor-I from rice: in vitro and in vivo studies. BMC Biotech. 2011;11:37-47.
120.Dressman JB, Amidon GL, Reppas C, Shah VP. Dissolution testing as a prognostic tool for oral drug absorption:immediate release dosage forms. Pharm Res. 1998;15:11-22.
121.Iarmon CS, Phizackerlet PJR. The effects of starvation and re-feeding on glycogen metabolism in mouse tail skin. Biochem J. 1983;212:679-683.
122.Inagawa H, Kohchi C, Soma GI. Oral administration of Lipopolysaccharides for the prevention of various diseases: Benefit and usefulness. Anticancer Research. 2011;31:2431-2436.
123.Lin K, Wang A. UV mutagenesis in Escherichia coli K-12: Cell survival and mutation frequency of the chromosomal genes lacZ, rpoB, ompF, and ampA. J Experimental Microbiology and Immunology. 2001;1:32-46.
124.Martiniuk F, Bodkin M, Tzall S, Hirschhorn R. Identification of the base pair responsible for a α glucosidase allele with lower affinity for glycogen (GAA 2) and transient gene expression in deficient cells. Am J Hum Genet. 1990;47:440-445.
125.Martiniuk F, Ellenbogen A, Hirschhorn K, Hirschhorn R. Further regional localization of the genes for human acid alpha glucosidase (GAA), peptidase D (PEPD) and alpha mannosidase (MANB) by somatic cell hybridization. Hum Genet. 1985;69:109-111.
126.Gomez-Lechon MJ, Ponsada X, Castell JV. A microassay for measuring glycogen in 96 well cultured cells. Anal Biochem. 1996;236:296-301.
127.Eskelinen EL, Illert AL, Tanaka Y, Schwarzmann G, Blanz J, von Figura K, Saftig P. Role of LAMP-2 in lysosome biogenesis and autophagy. Mol Biol Cell. 2002;13:3355-3368.
128.Eskelinen EL, Schmidt CK, Neu S, Willenborg M, Fuertes G, Salvador N, Tanaka Y, Lullmann-Rauch R, Hartmann D, Heeren J, von Figura K, Knecht E, Saftig P. Disturbed cholesterol traffic but normal proteolytic function in LAMP-1/LAMP-2 double-deficient fibroblasts. Mol Biol Cell. 2004;15:3132-3145.
129.Koike M, Shibata M, Waguri S, Yoshimura K, Tanida I, Kominami E, Gotow T, Peters C, von Figura K, Mizushima N, Saftig P, Uchiyama Y. Participation of autophagy in storage of lysosomes in neurons from mouse models of neuronal ceroid-lipofuscinoses (Batten disease). Am J Pathol. 2005;167:1713-1728.
130.Tanaka Y, Guhde G, Suter A, Eskelinen EL, Hartmann D, Lullmann-Rauch R, Janssen P, Blanz J, von Figura K, Saftig P. Accumulation of autophagic vacuoles and cardiomyopathy in LAMP-2-deficient mice. Nature. 2000;406:902-6.
131.Theeuwes F, Yum SI. Principles of the design and operation of generic osmotic pumps for the delivery of semisolid or liquid drug formulations. Ann Biomed Eng. 1976;4:343-353.
132.Cao Y, Espinola JA, Fossale E, Massey AC, Cuervo AM, Macdonald ME, Cotman S.L. Autophagy is disrupted in a knock-in mouse model of juvenile neuronal ceroid lipofuscinosis. J Biol Chem. 2006;29:20483-20493.
133.Raben N, Shea L, Hill V, Plotz P. Monitoring autophagy in lysosomal. Methods Enzymol. 2009;453:417-449.
134.Schmued LC, Stowerts CC, Scallet AC, Xu L. Fluoro-Jade C results in ultra high resolution and contrast labeling of degenerating neurons. Br Res. 2005;1035:24–31.
135.Bian G-L, Wei L-C, Shi M, Wang YQ, Cao R, Chen LW. Fluoro-Jade C can specifically stain the degenerative neurons in the substantia nigra of the 1-methyl-4-phenyl-1,2,3,6-tetrahydro pyridine-treated C57BL/6 mice. Br Res. 2007;1150:55–61.
136.Falk DJ, Todd AG, Lee S, Soustek MS, ElMallah MK, Fuller DD, Notterpek L, Byrne BJ. Peripheral nerve and neuromuscular junction pathology in Pompe disease. Hum Mol Genet. 2015;24:625–636.
137.Bourne D. A First Course in Pharmacokinetics and Biopharmaceutics/Biopharmaceutics. 2010; www.boomer.org/c/p1/.
138.loannou Y, Zeidner K, Gordon R, Desnick R. Fabry Disease: preclinical studies demonstrate the effectiveness of b-galactosidase a replacement in enzyme-deficient mice. Am J Hum Genet. 2001;68:14-25.
139.Schinkel A, Mayer U, Wagnenaar E, Mol C, Van Deemter L, Smit J, Van Der Valk M, Voordou A, Spits H, Van Telligen O, Zijlmans M, Fibbe W, Borst P. Normal viability and altered pharmacokinetics in mice lacking mdr1-type (drug-transporting) P-glycoproteins. Proc Natl Acad Sci. 1997;94:4028-4033.
140.van Tellingen O, Beijnen J, Verweij J, Scherrenburg E, Nooijen W, Sparreboom A. Rapid esterase-sensitive breakdown of polysorbate 80 and its impact on the plasma pharmacokinetics of docetaxel and metabolites in mice. Clin Cancer Res. 1999;5:2918-2924.
141.Maga JA, Zhou J, Kambampati R, Peng S, Wang X, Bohnsack RN, Thomm A, Golata S, Tom P, Dahms NM, Byrne BJ, LeBowitz JH. Glycosylation-independent lysosomal targeting of acid α-glucosidase enhances muscle glycogen clearance in pompe mice. J Biol Chem. 2013;288:1428-38.
142.Zhu Y, Li X, McVie-Wylie A, Jiang C, Thurberg BL, Raben N, Mattaliano RJ, Cheng SH. Carbohydrate-remodelled acid α-glucosidase with higher affinity for the cation-independent mannose 6-phosphate receptor demonstrates improved delivery to muscles of Pompe mice. Biochem J. 2005;389:619–628.
143.McVie-Wylie AJ, Lee KL, Qiu H, Jin X, Do H, Gotschall R, Thurberg BL, Rogers C, Raben N, O'Callaghan M, Canfield W, Andrews L, McPherson JM, Mattaliano RJ. Biochemical and pharmacological characterization of different recombinant acid α-glucosidase preparations evaluated for the treatment of Pompe disease. Mol Genet Metab. 2008;94:448–455.
144.Tong PY, Kornfeld S. Ligand interactions of the cation-dependent mannose 6-phosphate receptor. Comparison with the cation-independent mannose 6-phosphate receptor J Biol Chem. 1989;264:7970–7975.
145.Fukuda T, Ewan L, Bauer M, Mattaliano RJ, Zaal K, Ralston E, Plotz PH, Raben N. Dysfunction of endocytic and autophagic pathways in a lysosomal storage disease. Ann Neurol. 2006;59:700-708.
146.Raben N, Schreiner C, Baum R, Takikita S, Xu S, Xie T, Myerowitz R, Komatsu M, van Der Meulen J, Nagaraju K, Ralston E, Plotz P. Suppression of autophagy permits successful enzyme replacement therapy in a lysosomal storage disorder-murine Pompe disease. Autophagy. 2010;6:1078-1089.
147.Lim J-A, Li L, Shirihai OS, Trudeau KM, Rosa Puertollano R. and Raben N. Modulation of mTOR
signaling as a strategy for the treatment of Pompe disease. EMBO Molecular Medicine. 2017;9:353-370.
148.Carroll B, Maetzel D, Maddocks OD, Otten G, Ratcliff M, Smith GR, Dunlop EA, Passos JF, Davies OR, Jaenisch R, Tee AR, Sarkar S and Korolchuk VI. Control of TSC2-Rheb signaling axis by arginine regulates mTORC1 activity. eLife. 2016;5:e11058-91.
149.Jung JW, Kim NS, Jang SH, Shin YJ, Yang MS. Production and characterization of recombinant human acid alpha-glucosidase in transgenic rice cell suspension culture. J. Biotechnol. 2016;226:44–53.
150.Jung J-W, Huy N-X, Kim H-B, Kim N-S, Giap DV, Moon-Sik Yanga,b, Production of recombinant human acid α-glucosidase with high-mannose glycans in gnt1 rice for the treatment of Pompe disease. J Biotechnology. 2017;249:42–50.
151.Hashizume F, Hino S, Kakehashi M, Okajima S, Nadano D, Aoki N, Matsuda T. Development and evaluation of transgenic rice seeds accumulating a type II-collagen tolerogenic peptide. Transgenic Res. 2008;17:1117-1129.
152.Keum YS, Khor TO, Lin W, Shen G, Kwon K H, Barve A, Li A, Koug AN. Pharmacokinetics and pharmacodynamics of broccoli sprouts on the suppression of prostate cancer in transgenic adenocarcinoma of mouse prostate(TRAMP) mice: implication of induction of Nrf2, H0-1 and apoptosis and the suppression of Akt-dependent kinase pathway. Pharma Res. 2009;26:2324-2331.
153.Lamphear BJ, Streatfield SJ, Jilka JM, Brooks CA, Barker DK, Turner DD, Delaney DE, Garcia M, Wiggins B, Woodard SL, Hooda EE, Tizard IR, Lawhorn B, Howard JA. Delivery of subunit vaccines in maize seed. J Contr Release. 2002;85:169-180.
154.Ning T, Xie T, Qiu Q, Yang W, Zhou S, Zhou L, Zheng C, Zhu Y, Yang D. Oral administration of recombinant human granulocyte macrophage colony stimulating factor expressed in rice endosperm can increase leukocytes in mice. Biotech Lett. 2008;30:1679-1686.
155.Pena Ramırez YJ, Tasciotti E, Gutierrez-Ortega A, Donayre Torres AJ, Olivera Flores MT, Giacca M, Gomez Lim MA. Fruit-specific expression of the human immunodeficiency virus type 1 Tat gene in tomato plants and Its immunogenic potential in mice. Clin Vaccine Immunol. 2007;14:685-692.
156.Sardana RK, Alli Z, Dudani A, Tackaberry E, Panahi M, Narayanan M, Ganz P, Altosaar I. Biological activity of human granulocyte-macrophage colony stimulating factor is maintained in a fusion with seed glutelin peptide. Transgenic Res. 2002;11:521-531.
157.Streatfield SJ, Lane JR, Brooks CA, Barker DK, Poage ML, Mayor JM, Lamphear BJ, Drees CF, Jilka JM, Hood EE, Howard JA. Corn as a production system for human and animal vaccines. Vaccine. 2003;21:812-815.
158.Suzuki K, Kaminuma O, Yang L, Takai T, Mori A, Umezu-Goto M, Ohtomo M, Ohmachi Y, Noda Y, Hirose S, Okumura K, Ogawa H, Takada K, Hirasawa M, Hiroi T, Takaiwa F. Prevention of allergic asthma by vaccination with transgenic rice seed expressing mite allergen: induction of allergen-specific oral tolerance without bystander suppression. Plant Biotechnology Journal. 2011;9:982-990.
159.Tackaberry ES, Dudani AK, Prior F, Tocchi M, Sardana R, Altosaar I, Ganz PR. Development of biopharmaceuticals in plant expression systems: cloning, expression and immunological reactivity of human cytomegalovirus glycoprotein B (UL55) in seeds of transgenic tobacco. Vaccine. 1990;17:3020-3029.
160.Takagi H, Hiroi T, Yang I, Tada Y, Yuki Y, Takamura K, Ishimitsu R, Kawauchi H, Kiyono H, Takaiwa F. A rice-based edible vaccine expressing multiple T cell epitopes induces oral tolerance for inhibition of Th2-mediated IgE responses. Proc Natl Acad Sci. 2005;102:17525-17530.
161.Takagi H, Hiroi T, Yang L, Takamura K, Ishimitsu R, Kawauchi H, Takaiwa F. Efficient induction of oral tolerance by fusing cholera toxin B subunit with allergen-specific T-cell epitopes accumulated in rice seed. Vaccine. 2008;26:6027-6030.
162.Takagia H, Hiroi T, Hirosea S, Yanga L, Takaiwaa F. Rice seed ER-derived protein body as an efficient delivery vehicle for oral tolerogenic peptides. Peptides. 2009;31:1421-1425.
163.Takaiwa F. Update on the use of transgenic rice seeds in oral immunotherapy. Immunotherapy. 2013;5:301-12.
164.Wu J, Yu L, Li L, Hu J, Zhou J, Zhou X. Oral immunization with transgenic rice seeds expressing VP2 protein of infectious bursal disease virus induces protective immune responses in chickens. Plant Biotech J. 2007;5:570-578.
165.Xie T, Qiu Q, Zhang W, Tingting Ning T, Yang W, Zheng C, Wanga C, Zhu Y, Yang D. A biologically active rhIGF-1 fusion accumulated in transgenic rice seeds can reduce blood glucose in diabetic mice via oral delivery. Peptides. 2008;29:1862-1870.
166.Yamada Y, Nishizawa K, Yokoo M, Zhao H, Onishi K, Teraishi M, Utsumi S, Ishimoto M, Yoshikawa M. Anti-hypertensive activity of genetically modified soybean seeds accumulating novokinin. Peptides. 2008;29:331-337.
167.Yang L, Kajiura H, Suzuki H, Hirose S, Fujiyama K, Takaiwa F. Generation of a transgenic rice seed-based edible vaccine against house dust mite allergy. Bioch Biophys Res Comm. 2008;365:334-339.
168.Yang L, Tada Y, Yamamoto MP, Zhao H, Yoshikawa M, Takaiw F. A transgenic rice seed accumulating an anti-hypertensive peptide reduces the blood pressure of spontaneously hypertensive rats. FEBS Lett. 2006;580:3315-3320.
169.Yang L, Wakasa Y, Takaiwa F. Biopharming to increase bioactive peptides in rice seed. JAOAC International. 2008;91:957-66.
170.Zimmermann J, Saalbach I, Jahn D, Giersberg M, Haehnel, S, Wedel J, Macek J, Zoufal K, Glunder G, Falkenburg D, Kipriyanov SM. Antibody expressing pea seeds as fodder for prevention of gastrointestinal parasitic infections in chickens. BMC Biotech. 2009;9:79-101.
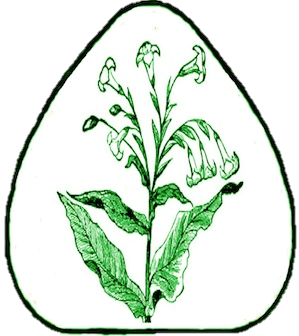
Comentarios